It would not be untrue, though perhaps unwise, to say to a burly coal miner ‘your coal is full of holes’. Indeed, a fundamental property which makes coal such a special and unique material – and has implications for coal properties ranging from not just methane holding capacity but also activated carbon and liquefaction – are these ‘holes’, or pores as they are more professionally referred to.
It is generally accepted that there are two key parts to the porosity system in coal: large fractures and much smaller pores (Clarkson and Bustin, 1999; Harpalani and Chen, 1997; Rodrigues and Lemos de Sousa, 2002; Cui et al., 2004). Within the smaller pore system it is recognised that three size classes of pore may exist (Şenel et al., 2001; Gan et al., 1972):
- Macropores, >50 nm in diameter
- Mesopores, 2 – 50 nm in diameter, and
- Micropores, <2 nm in diameter.
Interestingly, Yao et al. (2008) report that pores may follow a fractal distribution, being primarily influenced by the presence of natural surface area irregularities such as original plant pores and mineral matter. It has been argued that the size distribution of pores is even more complex than a ‘simple’ bi- or trimodal distribution with porosity being influenced by a range of types and sizes of micro-fractures (Gamson et al., 1996; Li et al., 2003). Understanding the “dual porosity” system is important because it is core to understanding gas desorption and flow.
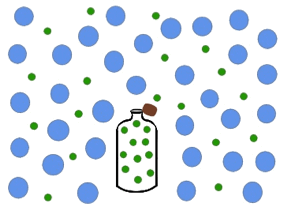
Diffusion of gas through the matrix from pore to pore is a process we see everyday, e.g. when you smell perfume.
It is well established that micropore volumes are associated with coals with high vitrinite content (Unsworth et al., 1989; Clarkson and Bustin, 1996; Bustin and Clarkson, 1998). Within an isorank series, Chalmers and Bustin (2007) found that bright lithotypes (i.e. vitrinite -rich) in Australian coals generally had greater pore volume than dull coals. Specifically they cite a positive correlation between the maceral telovitrinite and the volume of micropores. In contrast, in a study of New Zealand Eocene age coals, Mares et al. (2009) cite a negative correlation between telovitrinite and macroporosity. Chalmers and Bustin (2007) found that ‘reactive’ inertinite had similar pore architecture to vitrinite and thus similar methane holding potential. Coals with higher non-reactive inertinite have a greater abundance of macro- and mesoporosity as opposed to microporosity (Lamberson and Bustin, 1993; Clarkson and Bustin, 1996; Adeboye and Bustin, 2011). Interestingly, Chalmers and Bustin (2007) also found that in liptinite-rich coals (e.g. ‘boghead’ coals) methane is held as solution gas within the pores as opposed to being adsorbed on pore surfaces. This is an example of a depositional environment, albeit at one end of the depositional system spectrum, influencing pore type distribution in a CBM reservoir. Zhang et al. (2010) has also cite a correlation between micropore distribution and coal facies, though more work on this needs to be conducted.
Finally, lets return to the title question: how many holes does it take to fill the Albert Hall? I will admit this has been a conceit to get the reader to think about holes in a real-world setting (as
opposed to the sometimes-hard-to-envision environment of a reservoir). The real question, as it relates to coal, should not be ‘how many’ but what size. As is obvious – and I’m sure that the Beatles were aware of this when they wrote this line in their song “A Day in the Life” – the question can have an infinite number of answers: one big hole could fill the Albert Hall or an infinitely small number of holes could also do the job. However, if we were looking for holes (read these as ‘pores’) to ‘fill’ a coal, we’d be looking for the smaller versions in order to maximize the surface area for gas adsorption.
REFERENCES
Adeboye, O.O., Bustin, R.M., 2011. Variation of gas flow properties in coal with probe gas, composition and fabric: Examples from Western Canadian Sedimentary Basin. International Journal of Coal Geology, doi: 10.1016/j.coal.2011.06.015.
Ayers Jr., W.B., 2002. Coalbed gas systems, resources, and production and a review of contrasting cases from the San Juan and Powder River basins. AAPG Bulletin, 86: 1853-1890.
Bustin, R.M., Clarkson, C.R., 1998. Geological controls on coalbed methane reservoir capacity and gas content. International Journal of Coal Geology, 38: 3-26.
Ceglarsk-Stefańska, G., Brzóska, K., 1998. The effect of coal metamorphism on methane desorption. Fuel, 77: 645-648.
Ceglarsk-Stefańska, G., Zarębska, K., 2002. The competitive sorption of CO2 and CH4 with regard to the release of methane from coal. Fuel Processing Technology, 77-78: 423-429.
Chalmers, G.R.L., Bustin, R.M., 2007. On the effects of petrographic composition on coalbed methane sorption. International Journal of Coal Geology 69, 288-304.
Clarkson, C.R., Bustin, R.M., 1996. Variation in micropore capacity and size distribution with composition in bituminous coal of the Western Canadian Sedimentary Basin. Fuel 75, 1483-1498.
Clarkson, C.R., Bustin, R.M., 1999. The effect of pore structure and gas pressure upon the transport properties of coal: a laboratory modeling study. 2. Adsorption rate modeling. Fuel, 78: 1345-1362.
Close, J.C., 1993. Natural fractures in coal, in: Law, B.E. and Rice, D.D. (Eds.), Hydrocarbons from coal. American Association of Petroleum Geologists, Tulsa, Oklahoma, pp. 119-132.
Cui, X., Bustin, M., Dipple, G., 2004. Differential transport of CO2 and CH4 in coalbed aquifers: Implications for coalbed gas distribution and composition. International Journal of Coal Geology, 88: 1149-1161.
Dawson, G.K.W., Esterle, J.S., 2010. Controls on coal cleat spacing. International Journal of Coal Geology 82, 213-218.
Durucan, S., Ahsan, M., Shi, J.-Q., 2009. Matrix shrinkage and swelling characteristics of European coals. Energy Procedia, 1: 3055-3062.
Fick, A., 1855. On liquid diffusion. Philosophical Magazine, 10: 30-39.
Gamson, P., Beamish, B., Johnson, D., 1996. Coal microstructure and secondary mineralization: their effect on methane recovery, in: Gayer, R., Harris, I. (Eds.), Coalbed Methane and Coal Geology. Geological Society, London, Special Publication No. 109, pp. 165-179.
Gan, H., Nandi, S.P., Walker Jr., P.L., 1972. Nature of the porosity in American coals. Fuel, 51: 272-277.
Gray, I., 1987. Reservoir engineering in coal seams: Part 1. The physical process of gas storage and movement in coal seams. SPE Reservoir Engineering 2, 28-34.
Gu, F., Chalaturnyk, R.J., 2006. Numerical simulation of stress and strain due to gas sorption/desorption and their effects on in situ permeability of coalbeds. Journal of Canadian Petroleum Technology, 45: 1-11.
Gürdal, G., Yalçin, M.N., 2001. Pore volume and surface area of the Carboniferous coals from the Zonguldak basin (NW Turkey) and their variations with rank and maceral composition. International Journal of Coal Geology, 48: 133-144.
Harpalani, S., Chen, G., 1995. Estimation of changes in fracture porosity of coal with gas emission. Fuel, 74: 1491-1498.
Harpalani, S., Chen, G., 1997. Influence of gas production induced volumetric strain on permeability of coal. Geotechnical and Geological Engineering 15, 303-325.
Harpalani, S., Prusty, B.K., Dutta, P., 2006. Methane/CO2 sorption modeling for coalbed methane production and CO2Sequestration. Energy & Fuels, 20: 1591-1599.
Lamberson, M.N., Bustin, R.M., 1993. Coalbed methane characteristics of Gates Formation coals, northeastern British Columbia: Effect of maceral composition. AAPG Bulletin 77, 2062-2076.
Levine, J.R., 1996. Model study of the influence of matrix shrinkage on absolute permeability of coal bed reservoirs, in: Gayer, R. and Harris, I. (Eds.), Coalbed methane and coal geology, Geological Society Special Publication, No 109, 197-212.
Li, H., Ogawa, Y., Simada, S., 2003. Mechanism of methane flow through sheared coals and its role on methane recovery. Fuel, 82: 1271-1279.
Mares, T.E., Radlinski, A.P., Moore, T.A., Cookson, D., Thiyagarajan, P., Ilavsky, J., Klepp, J., 2009. Assessing the potential for CO2 adsorption in a subbituminous coal, Huntly Coalfield, New Zealand, using small angle scattering techniques. International Journal of Coal Geology, 77: 54-68.
Milewska-Duda, J., Duda, J., Nodzeñski, A., Lakatos, J., 2000. Absorption and adsorption of methane and carbon dioxide in hard coal and active carbon. Langmuir, 16: 5458-5466.
Moore, T.A. and Zarrouk, S.J., 2011. The origin and significance of gas saturation in coalbed methane plays: Implications for Indonesia. Proceedings, Indonesian Petroleum Association, 35th Annual Convention & Exhibition, May, Jakarta, Indonesia, Paper IPA11-G-195, not paginated.
Ozdemir, E., Morsi, B.I., Schroeder, K., 2003. Importance of volume effects to adsorption isotherms of carbon dixoide on coals. Langmuir, 19: 9764-9773.
Ozdemir, E., Schroeder, K., 2009. Effect of moisture on adsorption isotherms and adsorption capacities of CO2 on coals. Energy & Fuels, 23: 2821-2831.
Philibert, J., 2005. One and a half century of diffusion: Fick, Einstein, before and beyond. Diffusion Fundamentals, 2: 1-10.
Pillalamarry, M., Harpalani, S., Liu, S., 2011. Gas diffusion behavoir of coal and its impact on production from coalbed methane reservoirs. International Journal of Coal Geology 86, 342-348.
Radlinski, A.P., Mastalerz, M., Hinde, A.L., Hainbuchner, M., Rauch, H., Baron, M., Lin, J.S., Fan, L., Thiyagarajan, P., 2004. Application of SAX and SANS in evaluation of porosity, pore size and surface area of coal. International Journal of Coal Geology, 59: 245-271.
Radovic, L.R., Menon, V.C., Leon Y Leon, C.A., Kyotani, T., Danner, R.P., Anderson, S., Hatcher, P.G., 1997. On the porous structure of coals: Evidence for an interconnected but constricted micropore system and implications for coalbed methane recovery. Adsorption 3, 221-232.
Rice, D.D., 1993. Composition and origins of coalbed gas, in: Law, B.E. and Rice, D.D. (Eds.), Hydrocarbons from coal. American Association of Petroleum Geologists, AAPG Studies in Geology 38, Tulsa, Oklahoma, pp. 159-184.
Rodrigues, C.F., Lemos de Sousa, M.J., 2002. The measurement of coal porosity with different gases. International Journal of Coal Geology, 48: 245-251.
Şenel, I.G., Gürüz, A.G., Yücel, H., 2001. Characterization of pore structure of Turkish coals. Energy & Fuels, 15: 331-338.
St. George, J.D., Barakat, M.A., 2001. The change in effective stress associated with shrinkage from gas desorption in coal. International Journal of Coal Geology, 45: 105-113.
Unsworth, J.F., Flowler, C.S., Jones, L.F., 1989. Moisture in coal: 2. Maceral effects on pore structure. Fuel 68, 18-26.
Wang, G.X., Wei, X.R., Wang, K., Massarotto, P., Rudolph, V., 2010. Sorption-induced swelling/shrinkage and permeability of coal under stressed adsorption/dsorption conditions. International Journal of Coal Geology, 83:46-54.
Wang, X., 2006. Influence of coal quality factors on seam permeability associated with coalbed methane production. PhD Thesis, University of New South Wales, Sydney, Australia, 338 pp.
Wang, X., Ward, C., 2009. Experimental investigation of permeability changes with pressure depletion in relation to coal quality, International Coalbed Methane Symposium. University of Alabama, Tuscaloosa, Alabama, 31 pp.
Zarrouk, S.J., 2008. Reacting flows in porous media. VDM Verlag Dr Muller Aktiengesellshaft & Co. KG, Berlin.
Zarrouk, S.J., Moore, T.A., 2009. Preliminary model for enhanced coalbed methane (ECBM) through CO2 injection: Huntly Coalfield, New Zealand. International Journal of Coal Geology, 77: 153-161.
Zhang, S., Tang, S., Tang, D., Pan, Z., Yang, F., 2010. The characteristics of coal reservoir pores and coal facies in Liulin district, Hedong coal field of China. International Journal of Coal Geology 81, 117-127.
Zhou, Y., Zhou, L., 2009. Fundamentals of high pressure adsorption. Langmuir, 25: 13461-13466.
[this blog is an abridged version of: Moore, T. A., 2012, How many holes does it take to fill the Albert Hall? The importance of porosity in a coalbed methane reservoir: 36th Annual Convention & Exhibition, Indonesian Petroleum Association, Not paginated]
Comments are closed.